Low-temperature molecular ion spectroscopy / dynamics at RICE
We have developed a cryogenic electrostatic ion storage ring (RIKEN Cryogenic Electrostatic Ring : RICE) for experimental research on ion spectroscopy and reaction dynamics.
In electrostatic storage rings, charged particles are trapped and guided along a racetrack-like orbit by electrostatic potentials without magnetic fields or radio-frequency potentials. This apparatus has atractive properties such as non-selectively for ion mass, low purtabation of trapped ions, and strage of ions in the form of a beam. In our apparatus, we can store atomic and molecular ions over 1000 seconds due to the ultra-high vacuum condition (~10-10 Pa). Additionally, the internal states of molecular ions can be controled by radiational cooling effects due to the cryogenic enviorment (~ 4 K).
RICE is one of three cryogenic ion storage rings in the world. The development of RICE began in 2010, and first ion beam storage (Ne+) was achieved in 2014.
We plan to conduct spectroscopy and ion molecular collision experiments applying original setups such as : (a) Pretrap line, (b) Neutral atom beam, (c) Superfluid helium droplets, (d) Superconducting TES microcalorimeter. The scope of the experiments is not only singly charged cations but also anions, highly charged ions, cluster ions, and biomolecular ions. These experiments are aimed at applications for several fields of research such as interstellar chemistry, radiation biology and fundamental physics.
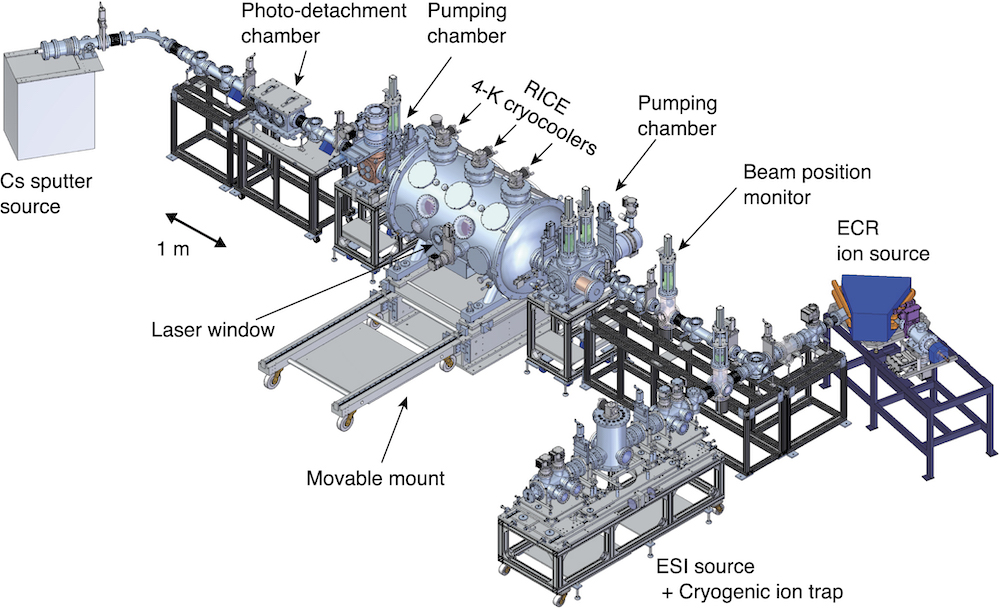
Rev. Sci. Instrum. 88, 033110 (2017)
(a) Pretrap line
The RIKEN Cryogenic Electrostatic (RICE) ion storage ring has opened up a new realm for the study of atomic and molecular ion spectroscopy and cooling dynamics with its ability to store ions for hundreds to thousands of seconds in an environment similar to that of the interstellar medium. Even at these conditions, however, the intrinsic relaxation times of ion excited states can be comparable to or longer than the allowable ring storage time. It is thus of interest to be able to inject ions which have been pre-cooled close to their ground state into the RICE ring for further study.
The so-called Pre-Trap Beamline was designed and built to fulfill this need for pre-cooled ions. The Pre-Trap Beamline consists of an ion source, a quadrupole mass filter, a cryogenic radio-frequency (RF) octupole ion trap, and a pulsed acceleration unit.
Two types of ion sources can currently be used with the Pre-Trap Beamline. One is an Electrospray Ionization (ESI) source and the other is a Laser Ablation Ion Source (LAIS). In the ESI source, ions are produced from a liquid solution containing the species of interest which is injected through a needle into the beamline. A high voltage of several kilovolts ionizes the species of interest within the droplets formed at the tip of the needle. ESI sources are well-known for their “soft” ionization, and are thus excellent at producing, for example, ions of large biomolecular species such as proteins. The LAIS produces ions through ablation of a target material with laser pulses. Light from a pulsed laser is focused onto the target material, producing ions of the material to be studied. The LAIS, while more violent in producing ions compared to the ESI source, is more versatile in its production of ions, only requiring that a solid target of the material be available.
Both the ESI source and LAIS produce ion bunches that contain the species of interest as well as molecules containing said species. In order to select for a specific ion species, a quadrupole mass filter is used. Here, an RF voltage is applied to the quadrupole structure which the ions pass through. For a given RF frequency, only a specific ion mass can pass through undisturbed; all other masses are filtered away. This allows for the production of pure ion bunches containing only the species of interest.
After the ion bunches are mass filtered, they are injected into the RF octupole ion trap. The ion trap is cooled down to 5.7 K using a cryochiller. In order to effectively cool the internal degrees of freedom of the ions, a helium buffer gas is introduced into the trap. Collisions with the helium buffer gas while in the trap cause de-excitation of excited states of the ions, leaving them closer to their ground state. As many as ~108 ions can be stored and cooled in the trap, yielding a sufficient quantity of pre-cooled ions to be injected into the RICE ring.
Ions extracted from the ion trap need to be accelerated to the storage energy of the RICE ring, which can range up 20 keV kinetic energy. To accomplish this, a pulsed acceleration unit is used. The acceleration unit is held at ground potential while the ion bunch travels towards it. Once the ion bunch is in the acceleration unit, it is quickly raised to the acceleration potential, e.g., 20 kV for 20 keV kinetic energy. Upon exiting the acceleration unit, the ion bunch is thus accelerated and of the appropriate storage energy to be used with the RICE ring.
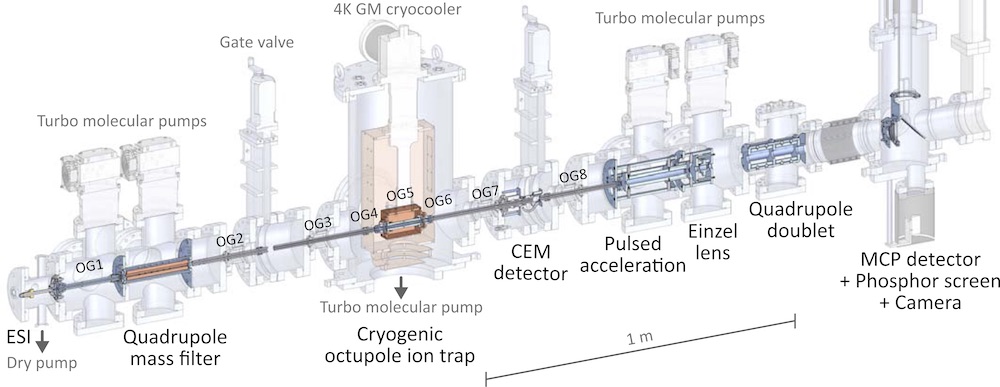
Rev. Sci. Instr. 89, 113110 (2018)
(b) Neutral atom beam
An interstellar cloud is a collection of gas and solid particles between stars. Those interstellar clouds with a particularly high density and mainly containing molecular hydrogen are called interstellar molecular clouds. Stars are born in interstellar molecular clouds. Although the density is high for an interstellar cloud, it is only about 102–104 particles per cm-3. These interstellar clouds are also very cold environments, with temperatures in the range of 10-100 K. Hence it looks like a very disadvantageous environment for molecular formation. However, with the progress of observational astronomy, more than 200 types of molecules have been found in the interstellar molecular clouds.
This fact suggests that there are active chemical reactions even at low temperatures. In particular, the reaction of ions with neutral atoms and molecules, so-called ion-molecule reaction, is considered to be one of the important low-temperature processes because the collision cross section increases on the low energy side.
Furthermore, chemical reactions in the low temperature regime clearly shows the individuality of the molecule, so that unique phenomena such as an increase in reaction rate due to quantum effects are likely to occur. There is the possibility that such an effect peculiar to low temperatures contributes to molecular formation in interstellar molecular clouds.
We aim to study the reaction dynamics of ion-molecule reactions by performing experiments that reproduce the interstellar molecular cloud environment using the RIKEN Cryogenic Electrostatic Ion Storage Ring (RICE). In particular, we are developing experiments to measure the reaction cross section of the ion-molecule reaction by colliding a hydrocarbon-based molecular ion trapped in RICE with a neutral atomic beam. In this way, the generation process of complex organic molecules (COMs), which has recently been attracting attention as a particularly important part of the development process of interstellar molecular clouds, can also be studied.
Since the ions trapped in RICE move with a uniform velocity distribution in the same direction, by injecting a neutral atomic beam at the same speed co-linearly, the relative collision speed between ions and neutrals can be very small. This method is called a merged beam method.
The 4 K environment provided by RICE not only enables long-time capture, which is indispensable for measurement experiments of reaction rate constants, but also enables vibrational and rotational energy levels of molecular ions to be controlled. From that, in addition to kinetic energy, it is possible to research the contributions of molecular ions to internal reactions.
We are currently developing a neutral atomic beam source to realize this experiment. Conditions to be satisfied by the neutral atomic beam source in this experiment are: (1) Neutral atomic velocity distribution is sufficiently narrow and beam angular spread is sufficiently small, (2) The relative velocity between the ion beam and the neutral beam can be accurately controlled and (3) The neutral atoms must be in their electronic ground state. Since the neutral atomic beam cannot be controlled by an electric or magnetic field, in order to satisfy (1) and (2), we choose the method of electron detachment from a beam of negative atomic ions. To satisfy (3), neutralization is performed by photodetachment using a laser.
In order to ensure enough photodetachment efficiency for the experiment, a Ti:Sa-based high-intensity semiconductor laser (5 kW) is used as the laser light source. We are also trying to increase the optical density by building an optical cavity. This neutral atomic beam source is in trial operation and will be connected to RICE in the future in order to conduct merged-beam experiments with ions beams stored in RICE.

(c) Superfluid helium droplet
Helium droplets are large liquid clusters of helium at 0.4 K. They can easily capture various molecular species and instantly cool the rotational and vibrational energies of the molecules down to 0.4 K. Additionally, the droplets are in the superfluid state, thus showing characteristic responses to the rotational motion of the captured molecules and to the relaxation process of the internal energies. This new project has started in 2014, aiming application of this helium droplet method to a wide range of molecular ions. With ions inside, the translational motion of the droplets can be controlled by conventional methods for charged particles. This project will be then focused on long-time scale molecular ion dynamics in helium droplets introduced into the RICE-Ring. Figure 5 illustrates the experimental scheme of the project. As the first step, we have started the development of a helium droplet machine for high efficiency production of molecular ions at 0.4 K.
Helium droplets are produced as a molecular beam from a high-pressure (> 1 MPa), low-temperature (< 20 K) nozzle of helium. After the expansion from a nozzle with a typical orifice diameter of 5 μm, helium atoms start to aggregate together to form large liquid clusters. Finally their temperature is lowered to 0.4 K by evaporation of the surface atoms. The resulting helium droplet beam is skimmed for molecular ion production at the downstream.
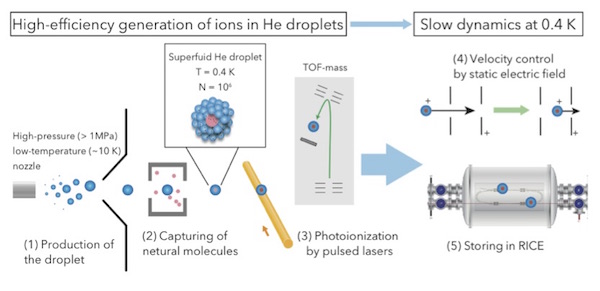
(d) Superconducting TES microcalorimeter
TES (Transition Edge Sensor) microcalorimeter is a highly sensitive thermal sensor that measures energy deposition via the increase in the resistance of a superconducting thin film that is biased within the sharp phase transition between the normal and superconducting phases. The TES microcalorimeter operated at cryogenic temperatures as low as 0.1 K resulting in ultra-low noise has excellent energy resolution, and is sensitive to all types of particles that can be converted to heat. In recent years, it has been rapidly developing as a single photon detector for energy decomposition from infrared to gamma rays.
In this laboratory, we promote the application of TES beam experiments through joint research with international superconducting detector teams such as the National Institute of Standards and Technology (NIST) and researchers in the field of astrophysics and nuclear physics. We are conducting a high-resolution X-ray spectroscopy experiments at a large accelerator facility using the most advanced multi-pixel TES as shown in below: “Muon atomic spectroscopy research using superconducting TES microcalorimeter“.
On the other hand, in RICE, TES is applied to the detection of “particles” rather than photons. In low-energy collision experiments, the most reliable way to identify a generated fragment is to measure its mass. However, if the product molecule is neutral, ordinary mass spectrometry cannot be applied without charge by ionization. In collision experiments in which the generated neutral molecule has almost the same velocity as the ion before the reaction, it is possible to identify the generated molecular species (mass measurement) by measuring the translational energy with high accuracy. It has only kinetic energy of 10 keV. For example, in semiconductor detectors, energy is dropped at the outermost surface (Si thickness is 0.1 μm or less) and does not reach the depletion layer. Conventionally, MCP (micro-channel plate), which has a relatively high detection efficiency, has been used, but there is essentially no energy discrimination capability, and there has been a long-awaited technological innovation for energy measurement of low-energy neutral particles.
TES is sensitive enough to low-energy incident neutral molecules that drop energy on the absorber surface, making it an ideal detector with high energy resolution. However, low energy neutral molecules, unlike X-rays, have low transmittance, so it is necessary to remove the thermal radiation shielding windows placed on each cooling stage (50mK, 3K, 50K). Fortunately, RICE has a low-temperature storage ring cooled to 4K, so it works well with the cryogenic detector TES and can minimize the effects of thermal radiation without windows. By applying TES as a superconducting molecule detector for the first time in the world and establishing a new high-resolution mass spectrometer, we can measure the mass of a neutral molecule in the final state that has not had an appropriate observation means until now.
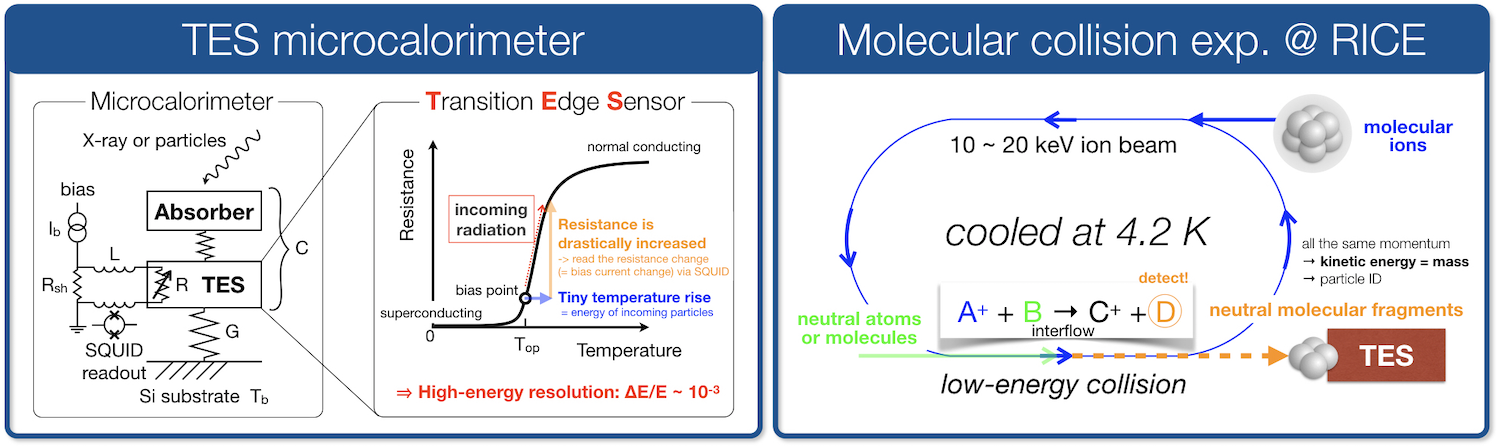
Fast highly charged heavy ion spectroscopy / dynamics via RCE method
When a fast ion beam is injected into a crystal, the ion feels the periodic field by atoms in the crystal as a temporally oscillating field. If the frequency of such filed matches the internal structure of the ion, the ion is excited. Such phenomenon is called Resonant Coherent Excitation, or RCE. RCE is one of the important techniques in X-ray region, where few coherent light source is available.
The experimental procedure is briefly summarized below. First we inject a collimated ion beam to a Silicon crystal. The Si crystal is mounted on a goniometer, which enables precise control of the crystal angle. The frequency of the electric field felt by the injected ions varies with some parameters such as ion’s velocity, distance between lattice planes, and the crystal angle. We can adjust the crystal angle so that the frequency matches the transition frequency of the ion. We observe the excitation of the ion either 1. by measuring the change in the charge states of the ion due to the collisional ionization after the excitation or 2. by measuring the X-ray emitted due to deexcitation.
Our group employs RCE for 1. manipulating internal states of ions and 2. testing bound-state Quantum Electrodynamics (bound-state QED) via precision spectroscopy of heavy ions.
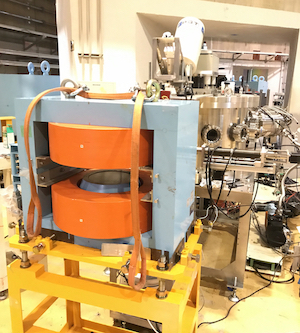
Low-temperature molecular spectroscopy with superfluid helium droplets
to be updated
Muonic atom X-ray spectroscopy using superconducting TES microcalorimeter
Negatively-charged muon can be bound by the Coulomb field of an atomic nucleus. This system, so-called muonic atom, is essentially a hydrogen-like atom. The muon is about 200 times more massive than the electron. The Bohr radius is therefore 200 times smaller, and thus the internal electric field strength is to be 40,000 times higher than that of normal atoms. The electric field is proportional to the cube of the atomic number Z. A heavier muonic atom has therefore an extremely strong electric field even larger than the Schwinger-critical-field strength, 1.32 ×1018 [V/m], where the electric field becomes nonlinear in quantum electrodynamics (QED).
This study aims to explore QED under extremely strong electric fields by high-precision X-ray spectroscopy of isolated highly-charged muonic atoms in a vacuum. In the de-excitation process from the highly excited state immediately after generation, the muon atom peels off the bound electrons that the atom originally had as Auger electrons, and eventually generates isolated hydrogen-like highly-charged muonic ions. While a low-density target is required to avoid rapid refilling of electrons into the highly-charged muonic atom from the surrounding atoms, it is experimentally difficult to efficiently stop muons in a low-density target due to their large momentum distribution via traveling pion decay. This results in insufficient x-ray yield with the conventional x-ray spectroscopy technology based on diffraction from Bragg crystals.
We aim to realize the high-resolution muonic atom X-ray spectroscopy with low-density gas target with a combination of the world highest intensity pulsed negative muon beam at J-PARC MUSE facility and an X-ray spectrometer based on a 240 pixel array of TES microcalorimeters (see the description above).

Highly-charged heavy ion laser spectroscopy / dynamics with EBIT
Recently, we have proposed a new experiment for laser spectroscopy and optical manipulation of highly charged ions (HCIs) trapped in an electron beam ion trap (EBIT). In the experiment, we have collaborated with Tokyo-EBIT Laboratory at the University of Electro-Communications.
In our proposal, we will irradiate a laser to trapped HCIs in the EBIT plasma to induce a de-excitation process from a metastable state in the HCIs. We expect that the method will realize not only an active control of energy states of HCIs in a plasma, but also a new method for precision spectroscopy of forbidden transitions between excited states of HCIs, which were difficult to measure with conventional experiments.
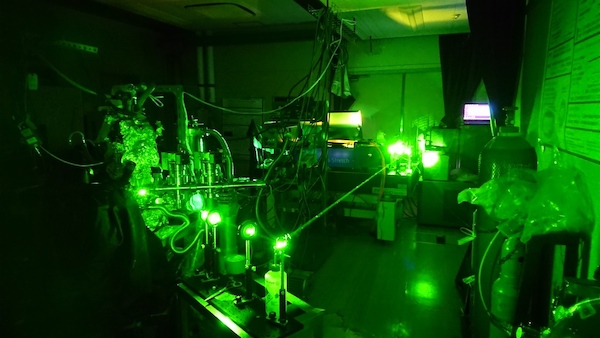
Laser spectroscopy of negative positronium ions
to be updated